Resting and Action Potentials of a Neuron
- Romit Chunduri
- Oct 3, 2021
- 5 min read
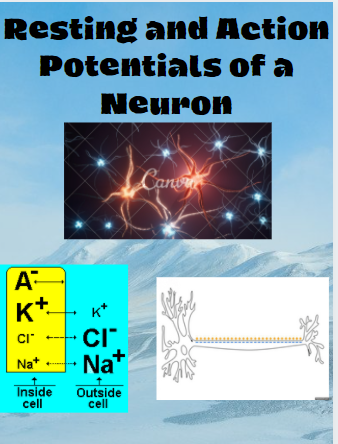
Hello everyone, and welcome to my third blog post. I have recently been inactive due to school work, but I am finally back and ready to educate. Today we will be talking in-depth about the resting and action potentials of a neuron!
Resting and Action Potentials of a Neuron
Nerves connect your brain to the rest of your muscles and organs. When you want to move your foot, your brain sends information to the muscles in your foot through your nerves. Your nerves, on the other hand, don't just say, "Foot, move." Instead, the nerves send numerous electrical impulses called action potentials to several muscles in your foot, allowing you to move your foot with extreme accuracy.
Neurons are a sort of cell that is solely responsible for the transport of information throughout the body. Neurons have a cell body that includes a nucleus and organelles, just like other cells. They do, however, have a few additional traits that allow them to be adept in transferring action potentials such as:
Axon: The axon, which is several times thinner than a strand of human hair, is where electrical impulses from the neuron travel away from the cell to be received by other neurons.
Axon Terminal: Axon terminals are tiny swellings present at the axon's terminal ends. Synapses with other neurons are frequently found there, and neurotransmitters are stored there to communicate with other neurons via these synapses.
Dendrites: Dendrites, which extend outward from the cell body and are specialized to receive chemical signals from the axon termini of other neurons, are found in most neurons. These signals are converted into little electric impulses by dendrites, which then transport them inside, toward the cell body.
Myelin Sheath: Myelin is a sheath-like insulating coating that forms around nerves, including those in the spinal cord and the brain. Protein and fatty components make up this substance. Electrical impulses can travel fast and efficiently along nerve cells thanks to the myelin coating.

Concentration Gradients: The differential in ion concentrations between the inside and outside of a neuron is called a concentration gradient. They are the key to how action potentials work. There would be a considerable concentration gradient if the concentration of positively charged ions outside the cell was higher than the concentration inside the cell. If there were more of one type of charged ion within the cell than outside, the same thing would happen. It makes no difference what the ion's charge is; both positively and negatively charged ions flow in the direction to balance or level out the gradient.
Action Potentials: Action potentials, which are electrical impulses that carry signals throughout your body, are nothing more than a momentary shift in the membrane potential of a neuron generated by ions flowing in and out of the cell. All gated sodium and potassium channels are closed in the resting state before an action potential arises. These gated channels differ from leaky channels in that they only open when an action potential is triggered. A signal from other cells connected to the neuron causes positively charged ions to flow into the cell body during an action potential. These ions enter the cell to depolarize it, but as they do so, they pass through channels that allow a substance known as a neurotransmitter to be released. As a result of their own action potential, these neurotransmitters are frequently released near dendrites. The ions cause depolarization by bringing the membrane potential close to zero. The polarity of the negative cell is reduced when positive ions flow into it. When the potential difference reaches a certain level, the reduced voltage causes hundreds of sodium gates in that membrane region to open briefly. As a result, sodium ions flood into the cell, depolarizing the membrane completely. This causes more voltage-gated ion channels in the adjacent membrane to open, causing a wave of depolarization to travel along the cell, which is the action potential.
Three main actions take place during an action potential:
Depolarization- This process reduces the polarity of the cell. Voltage-gated sodium channels in the axon closest to the cell body open as a result of the recently depolarized cell body cursing the positively charged sodium ions to enter the negatively charged axon and depolarize it. Despite the fact that this step is referred to as depolarization, the neuron goes past equilibrium and becomes positively charged due to the action potential passing through.
Repolarization- This process brings the cell back to its resting potential. The sodium channels' inactivation gates close, halting the inward flow of positive ions. The potassium channels open at the same time. Because there is significantly more potassium inside the cell than outside, when these channels open, more potassium exits than enters. This indicates that the cell gets deprived of positively charges ions causing it to return to its resting state.
Hyperpolarization- makes the cell's membrane potential more negative than its resting membrane potential. As the action potential passes through, it causes the potassium channels to be open for a longer period of time; this causes positive ions to exit the neuron. This means that the cell temporarily becomes more negatively charged than when it was at rest causing hyperpolarization. The sodium-potassium pump then works to restore the resting state while the potassium channels close.
The foundations of an action potential resided in the fact that it is either triggered or it isn’t. The always sends the same size action potential regardless of the message, but the distinguishing factor is how many times it is sent which determines the relative significance of the message. When sending action potentials, there is usually a time frame set in order for the cell to send these action potentials which is called the refractory period. This is the brief moment in which the cell stops sending signals and it’s generally around 1 millisecond. The refractory period allows cells to replenish neurotransmitters and help the cells last longer.
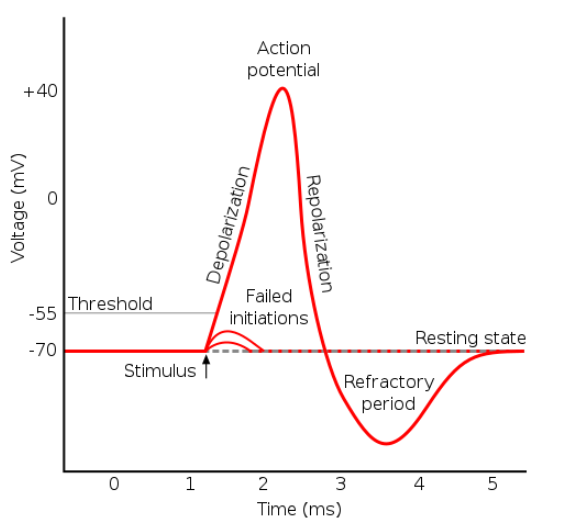
Resting Potentials:
The resting membrane potential is determined by the concentrations of ions inside and outside the cell. The resting membrane potential is dominated by the difference in the number of positively charged potassium ions inside and outside the cell. A neuron is said to be at rest when it is not sending a signal. A neuron is at rest when the inside of the neuron has a negative charge relating to its surroundings. Although the different ion concentrations try to balance out on both sides of the membrane, only some ions are able to pass through. At rest, Potassium ions are easily able to pass through while sodium and chloride ions find it more difficult. A neuron's resting membrane potential is around -70 mV (millivolt), which means that the inside of the neuron is 70 mV lower than the outside. At rest, there are more sodium ions outside the neuron while there are more potassium ions within.
That is it for today's blog, thank you for having the passion and willingness to learn the basics of the action and resting potentials within a neuron, as understanding these basics can help you comprehend neuroscience easier. Next time we will be continuing our neuroscience journey with information on neurotransmitters and receptors. As always, please reach out if there are any questions, and let me know in what ways I can improve my blogs to bring the most fun in neuroscience.
Comments